If you have pure creativity but you don’t know anything, it’s too bad. Sometimes it’s bad to know too much. Wolfgang Pauli complained about his lack of creativity and said, ‘I know too much!’ If you know too much, then you don’t have that fresh view which allows you to see the breakthrough idea.
Leon Max Lederman was born in New York City, the second son of Russian-Jewish immigrants. He studied chemistry at City College of New York, receiving his bachelor of science degree in 1943. Following three years in the army during World War II, he studied physics at Columbia University, earning his master’s in 1948 and his Ph.D. in 1951.
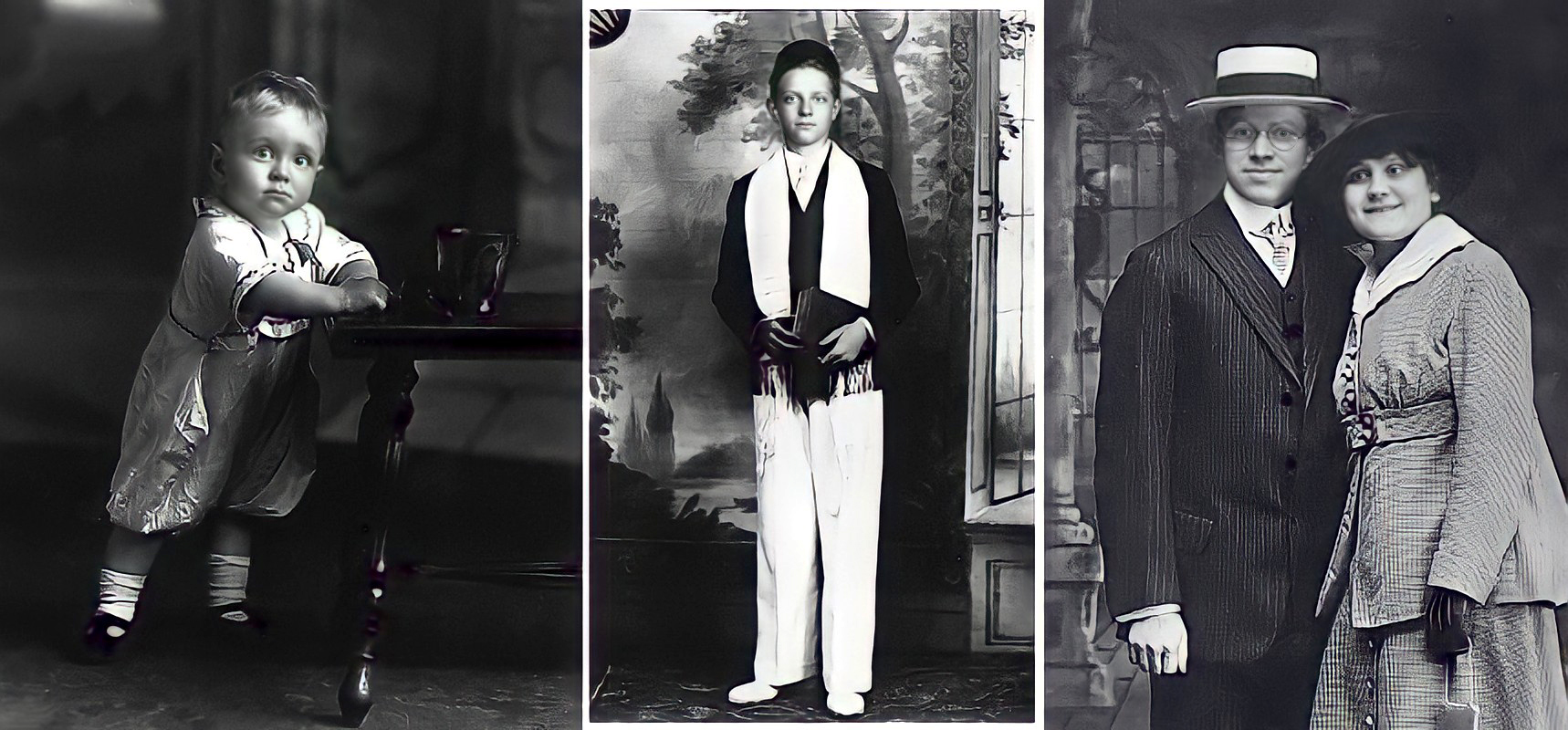
Leon Lederman stayed on at Columbia following his studies, for nearly 30 years, as the Eugene Higgins Professor and, from 1961 until 1979, as director of Nevis Laboratories in Irvington, the Columbia physics department center for experimental research in high energy physics. With colleagues and students from Nevis, he led an intensive and wide-ranging series of experiments that provided major advances in the understanding of “weak interactions,” one of the fundamental nuclear forces. In 1956, working with a Columbia team at the Brookhaven National Laboratory on Long Island, Lederman discovered a new particle, the long-lived neutral K-meson, which had been predicted from theory. Further research at Columbia demonstrated the non-conservation of parity during muon decay.
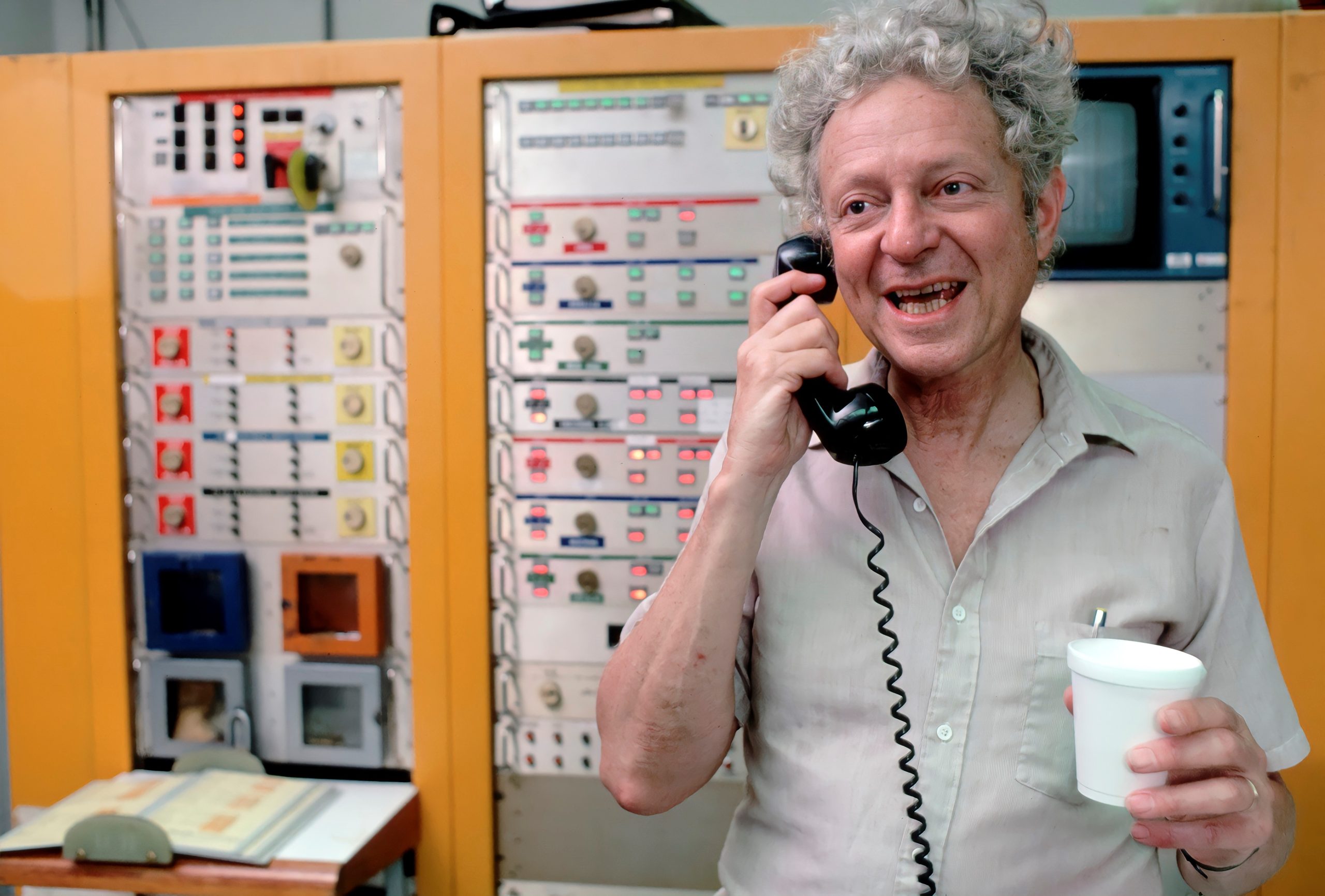
In the early 1960s, Lederman and his colleagues were preoccupied with neutrinos, ghostlike particles that pass through everything in the universe. At the time, only the electron neutrino was known, and the scientists wondered if they could find more types of neutrinos. Columbia’s Alternating Gradient Synchrotron (AGS), then the most powerful accelerator in the world, was capable of producing the beam needed to perform the necessary experiments. In 1962, Dr. Lederman, with his colleagues Melvin Schwartz and Jack Steinberger, succeeded in identifying the second such particle, the muon neutrino.
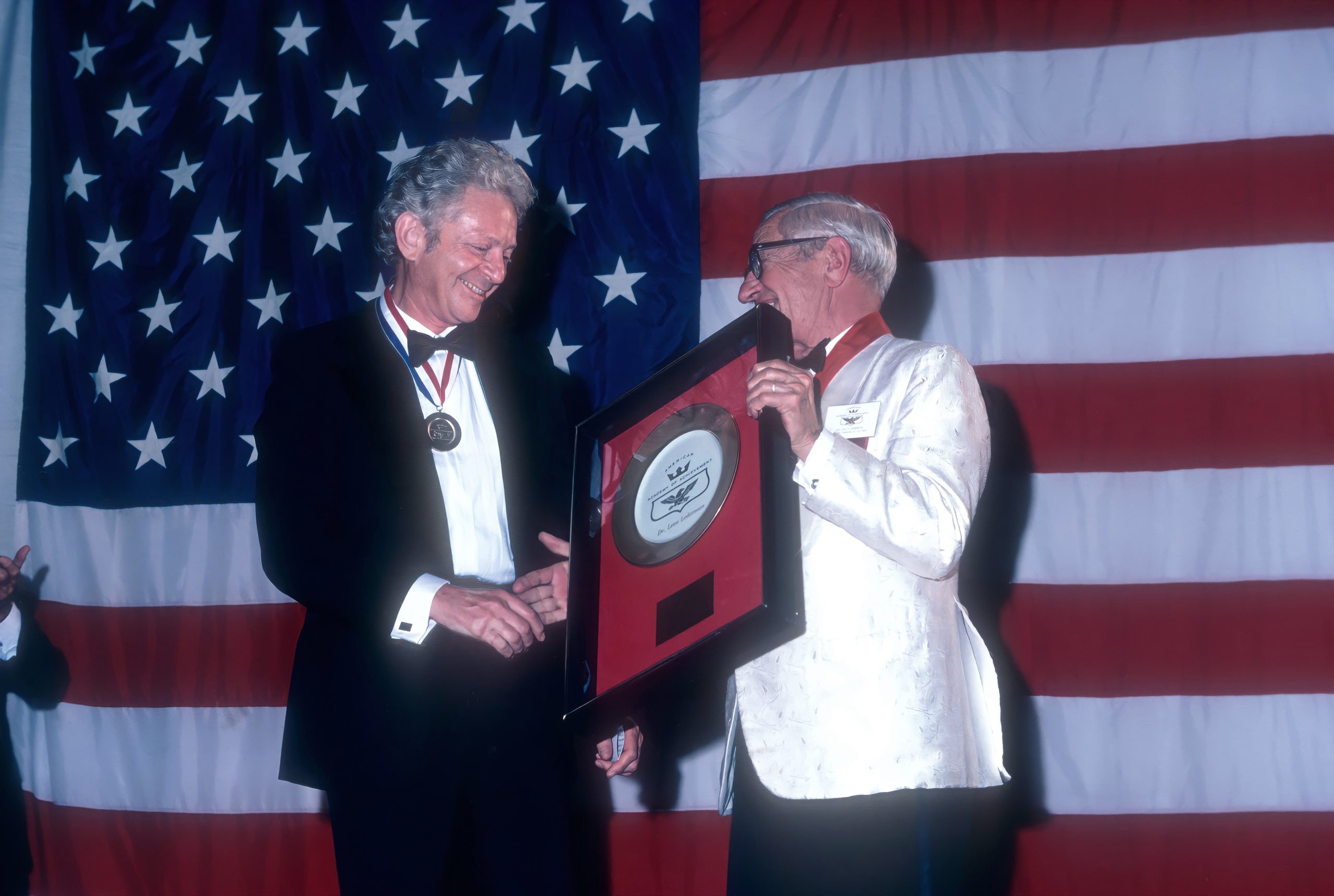
The experiment used a beam of the AGS’s energetic protons to produce a shower of pi mesons, which traveled 70 feet toward a 5,000-ton steel wall made of old battleship plates. On the way, they decayed into muons and neutrinos, but only the latter particles could pass through the wall into a neon-filled detector called a spark chamber. There, the impact of neutrinos on aluminum plates produced muon spark trails that could be detected and photographed — proving the existence of muon neutrinos.
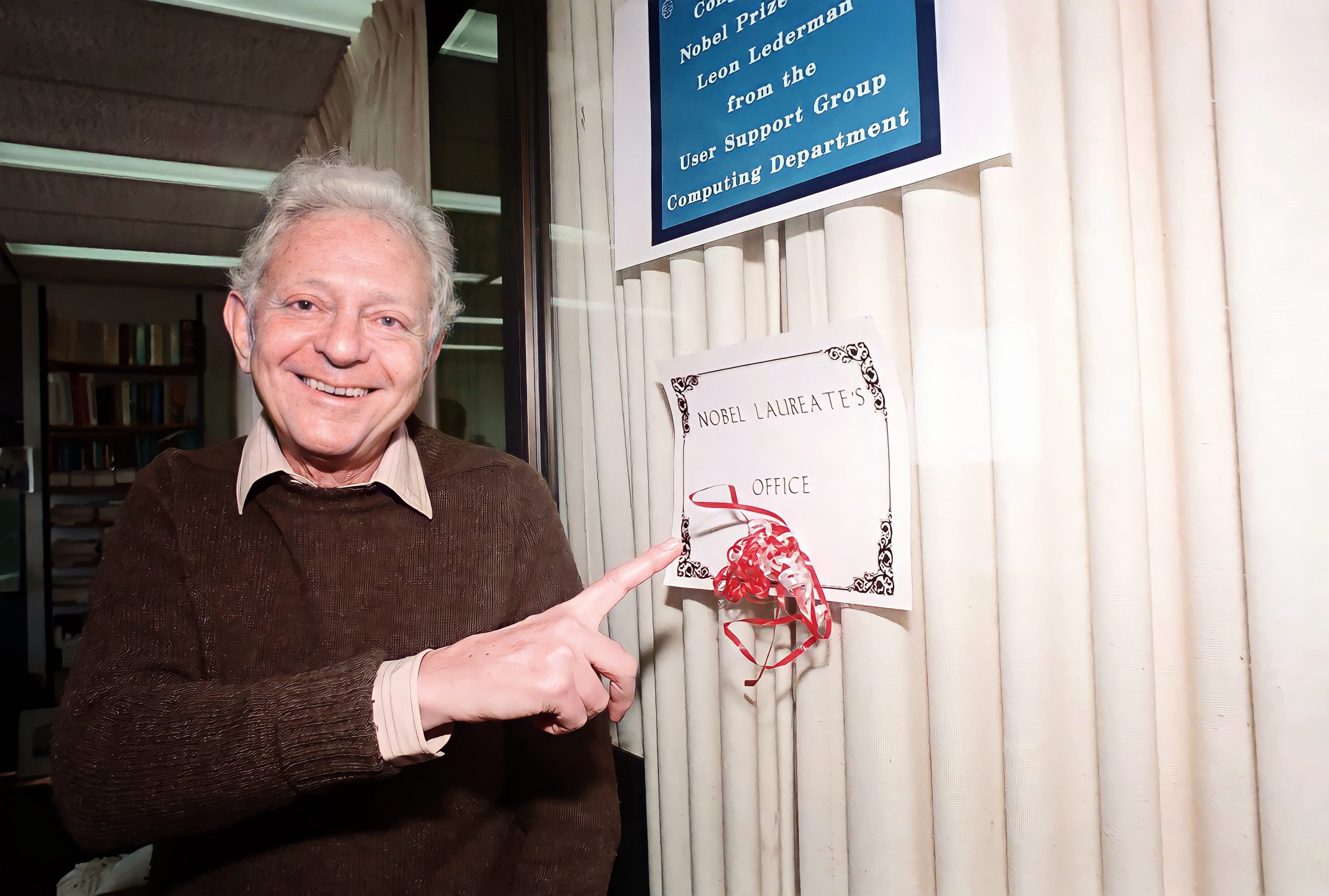
The experiment’s use of the first-ever neutrino beam paved the way for scientists to use these particles in research at the AGS and around the world, and eventually netted Lederman and his partners the Nobel Prize in Physics. Since the team’s work, neutrinos have been used as a way of analyzing everything from the structure of the atomic nucleus to the energy level of an exploding star, or supernova.
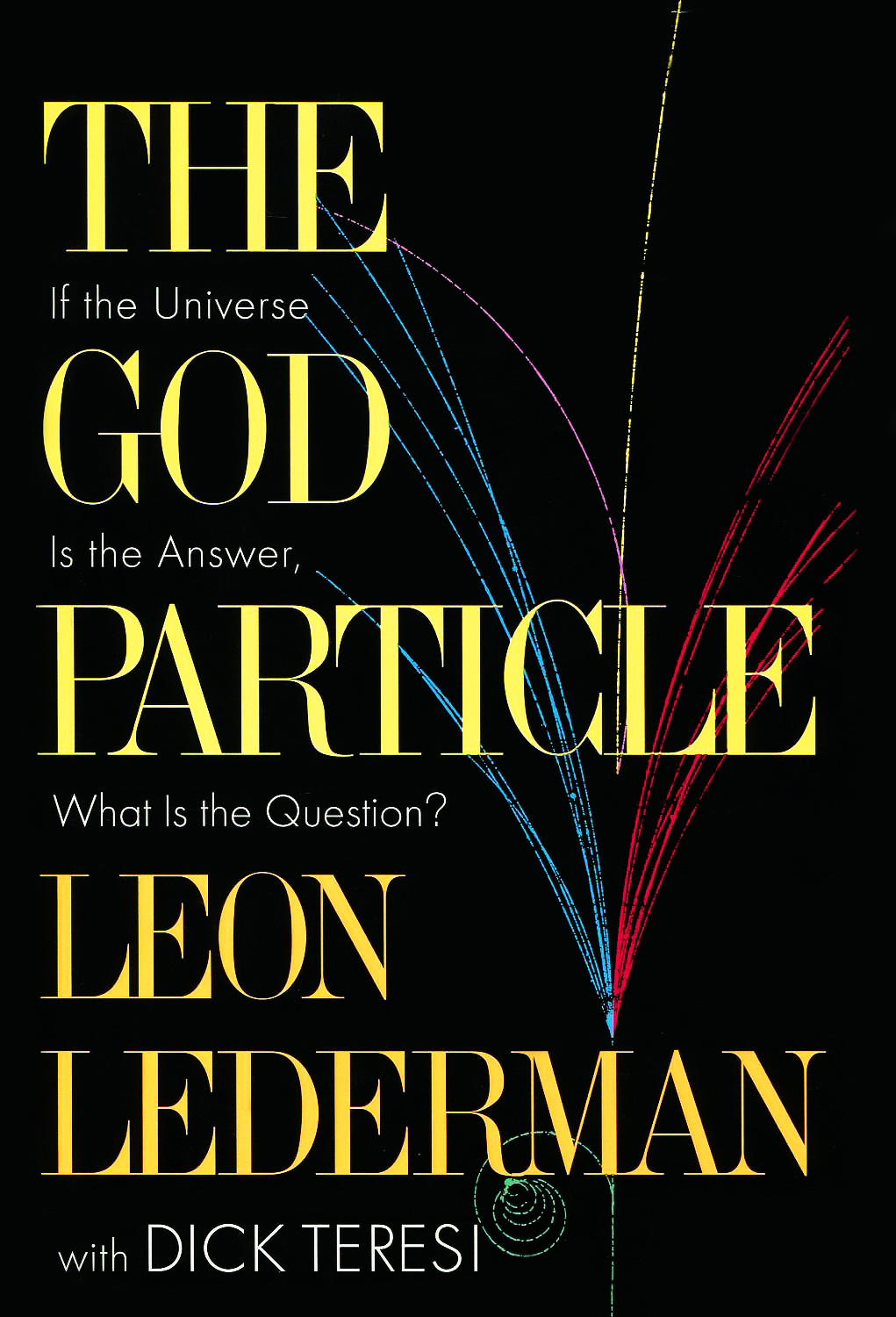
This early award-winning research in high energy physics brought Dr. Lederman into national science policy circles, and in 1963, he proposed the idea that eventually became the Fermi National Accelerator Laboratory in Batavia, Illinois.
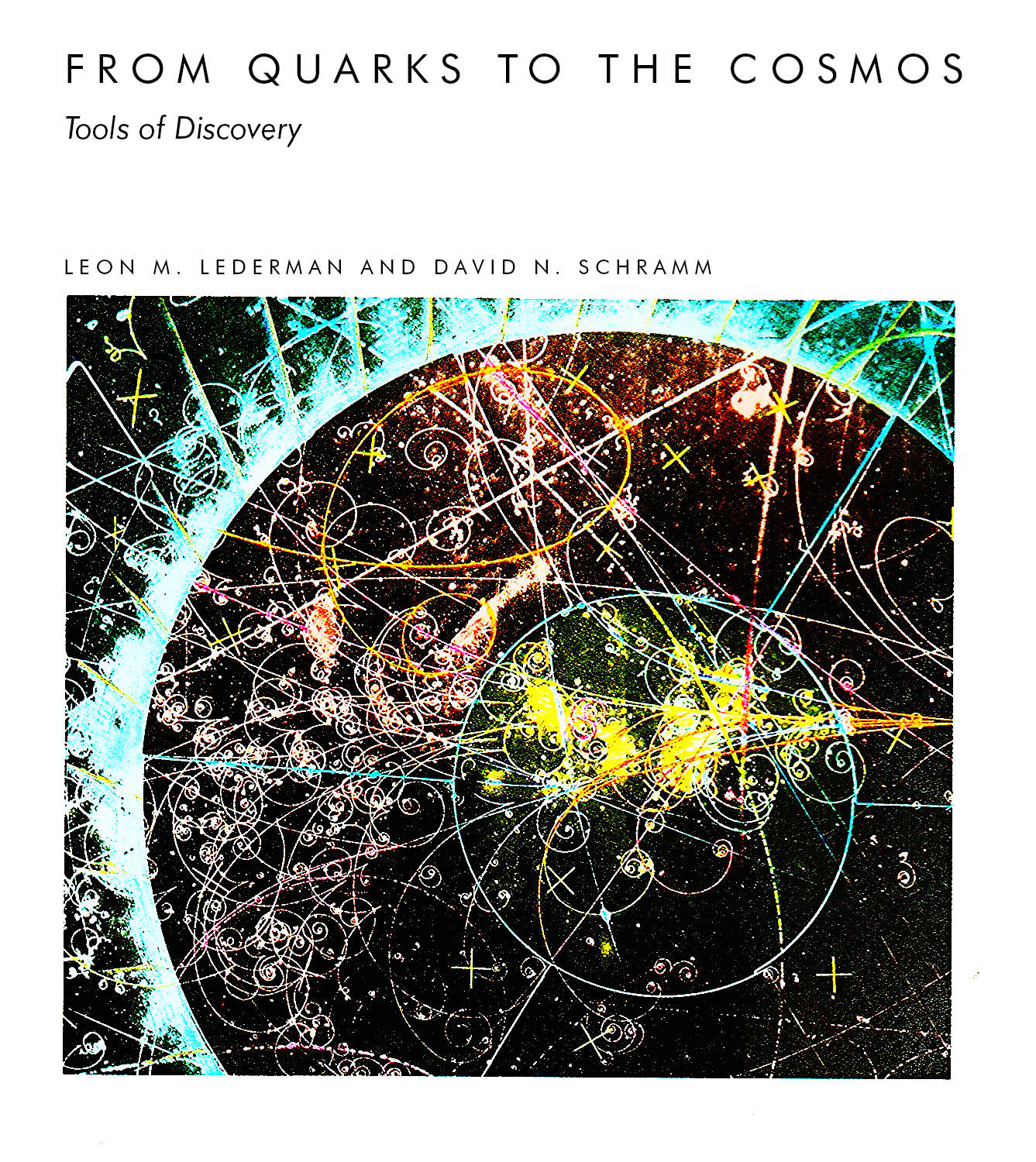
The design of ever more powerful accelerators, first at Brookhaven National Laboratory, enabled Lederman and his team to find the first antimatter particle in 1965. In 1977, Lederman led the team at Fermilab that discovered the subatomic particle known as the bottom quark. The following year, he was named director of the laboratory. By 1983, his administration had brought Fermilab into a position of international prominence with the construction of the world’s most powerful superconducting accelerator, the Tevatron.
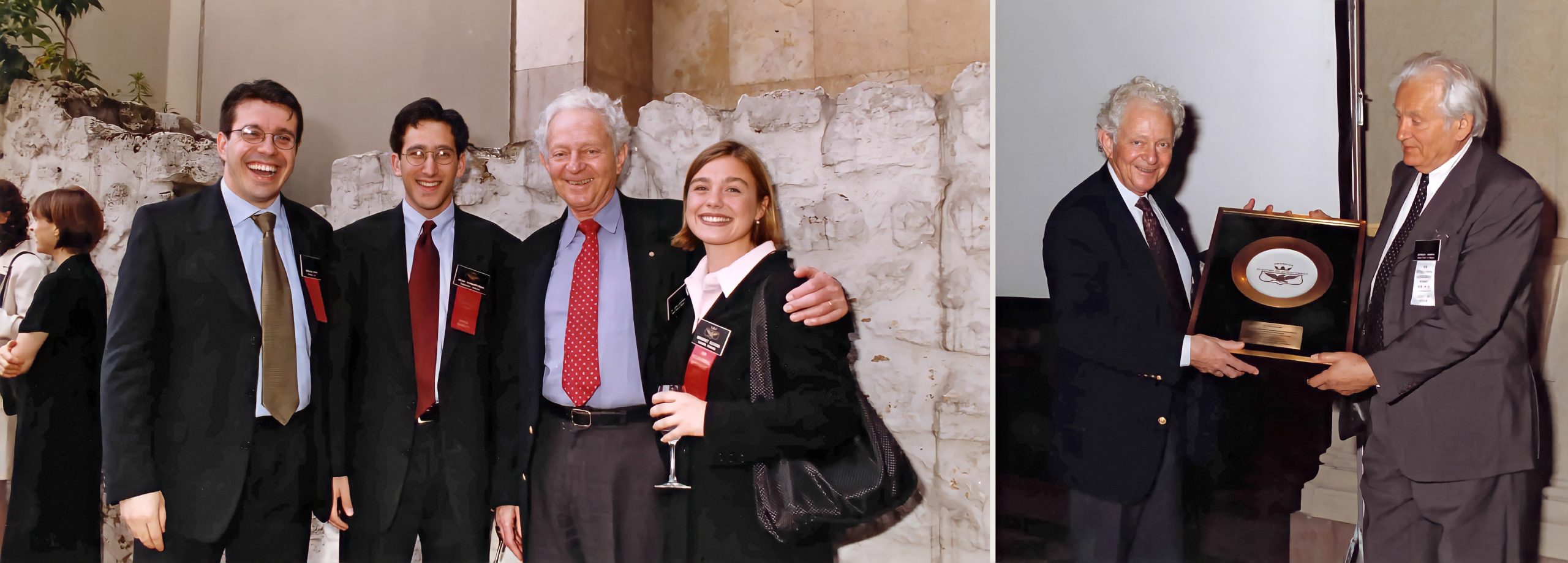
A convinced proponent of science education, Lederman opened Fermilab to countries not previously associated with high energy physics. During his term as director, Lederman also emphasized the importance of math and science education as outreach to neighboring communities. He initiated the Saturday Morning Physics lectures and subsequently founded the Friends of Fermilab.
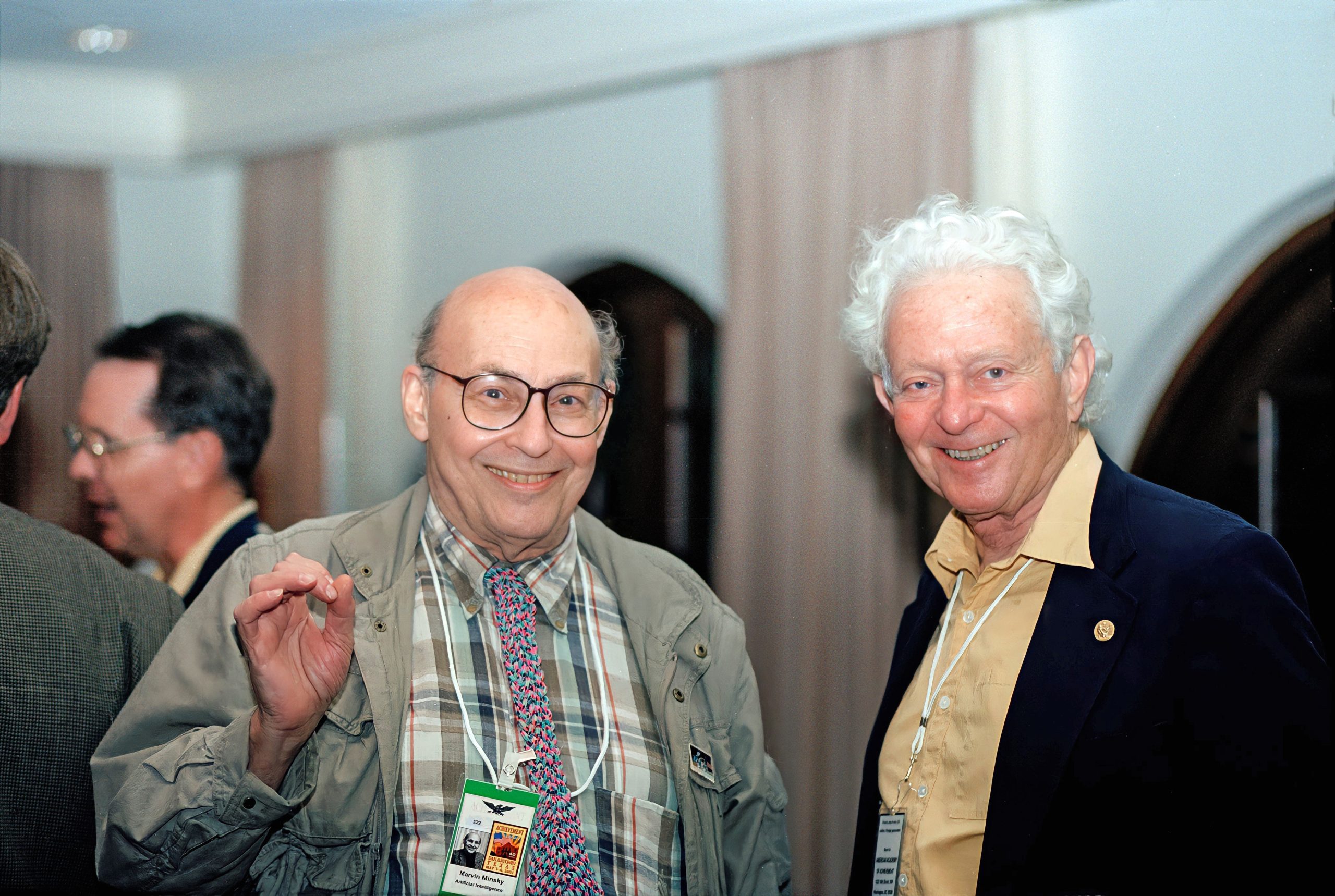
The 1988 Nobel Prize in Physics was awarded to Lederman and his partners, Schwartz and Steinberger, for “transforming the ghostly neutrino into an active tool of research.” Lederman used his share of the prize money to buy a vacation cabin in Idaho. In 1989, Dr. Lederman stepped down as director of Fermilab and assumed the title director emeritus. He then served as Frank L. Sulzberger Professor of Physics at the University of Chicago and pursued his increasing interest in the problems of science education in American schools. He founded the Illinois Mathematics and Science Academy, the first state-wide residence public school for gifted children, and the Teacher’s Academy of Mathematics and Science in Chicago.
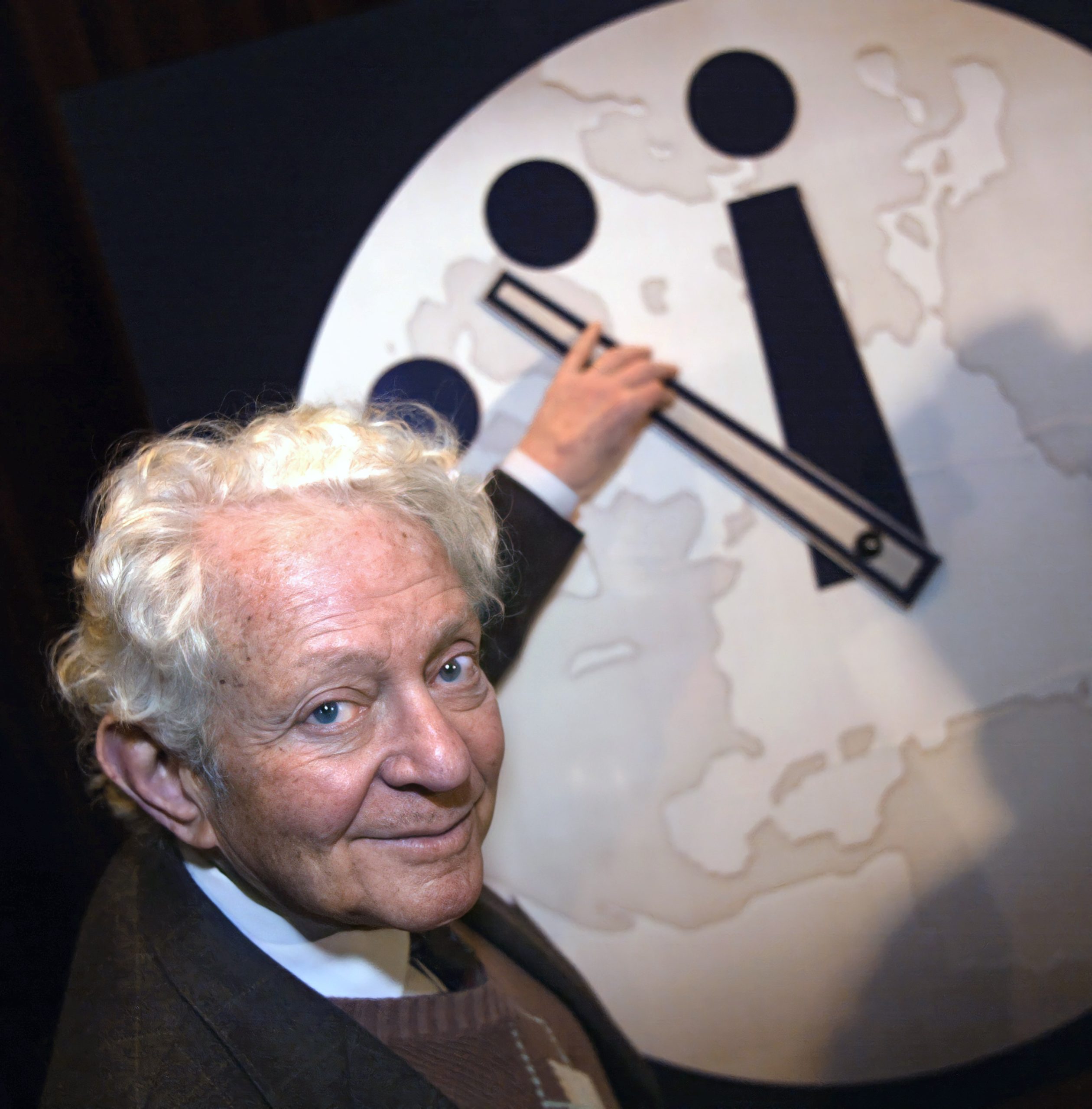
Dr. Lederman was Pritzker Professor of Physics at the Illinois Institute of Technology. He is also a member of the National Academy of Sciences and has received numerous awards besides the Nobel, including the National Medal of Science (1965), the Elliott Cresson Medal of the Franklin Institute (1976), and the Wolf Prize in Physics (1982). He is a past chairman and president of the American Association for the Advancement of Science. In 1993, he was awarded the Enrico Fermi Prize by President Clinton. He has served as founding member of the High Energy Physics Advisory Panel and the International Committee for Future Accelerators.
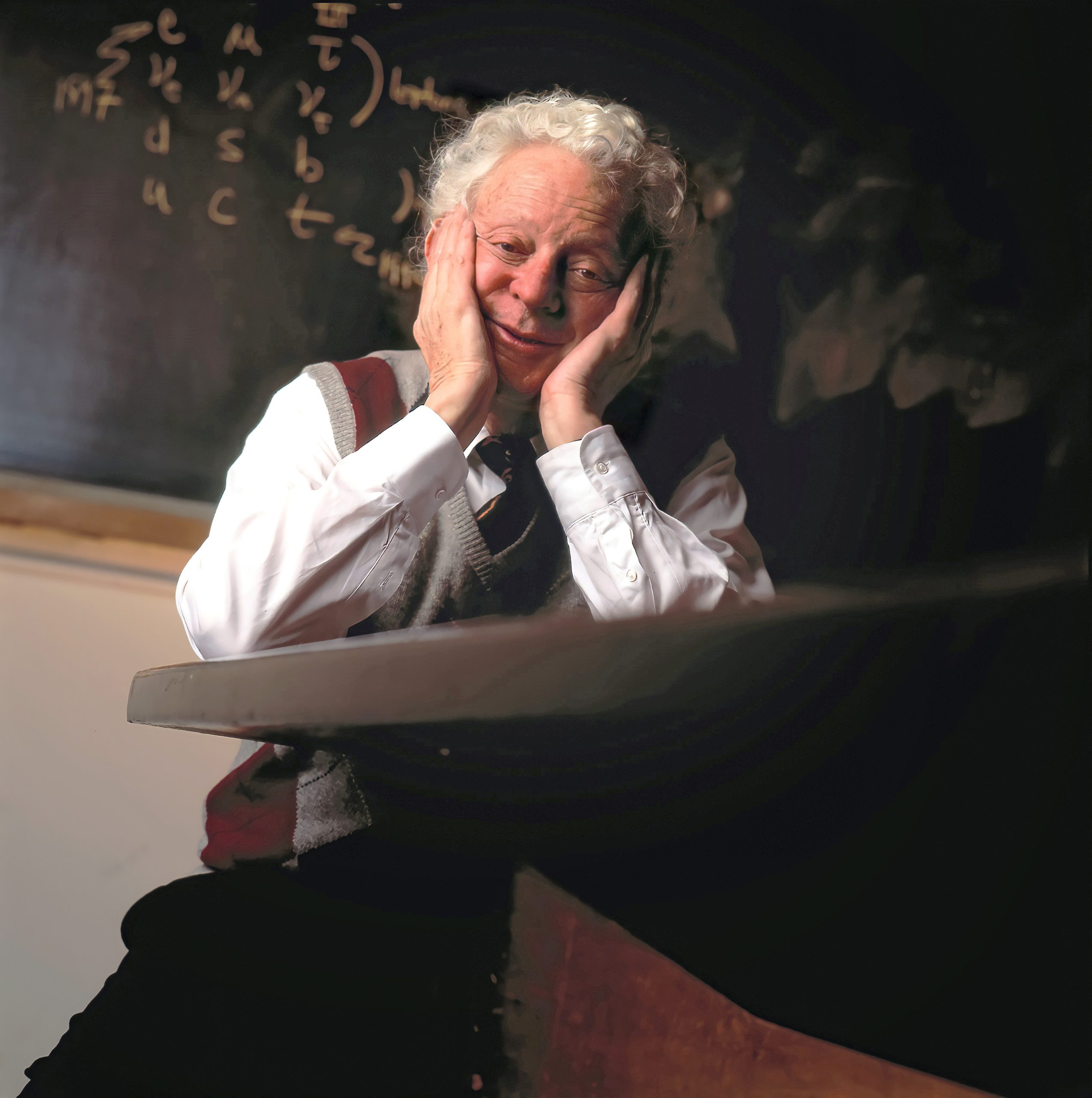
In 1994, researchers at Fermilab achieved an old goal of Dr. Lederman’s, detecting the top quark, the bottom quark’s elusive companion, which had escaped observation for the previous 17 years.
Leon Lederman’s publication list runs to 200 papers. He is co-author of the books The God Particle: If the Universe Is the Answer, What Is the Question? (1989, with Dick Teresi), From Quarks to the Cosmos: Tools of Discovery (1995, with David N. Schramm), Symmetry and the Beautiful Universe (2004) and Quantum Physics for Poets (2011), both co-authored by Christopher T. Hill. In these works, Lederman used humor, metaphor, and storytelling to delve into the mysteries of matter, discussing particle accelerators and the elusive “God particle.”
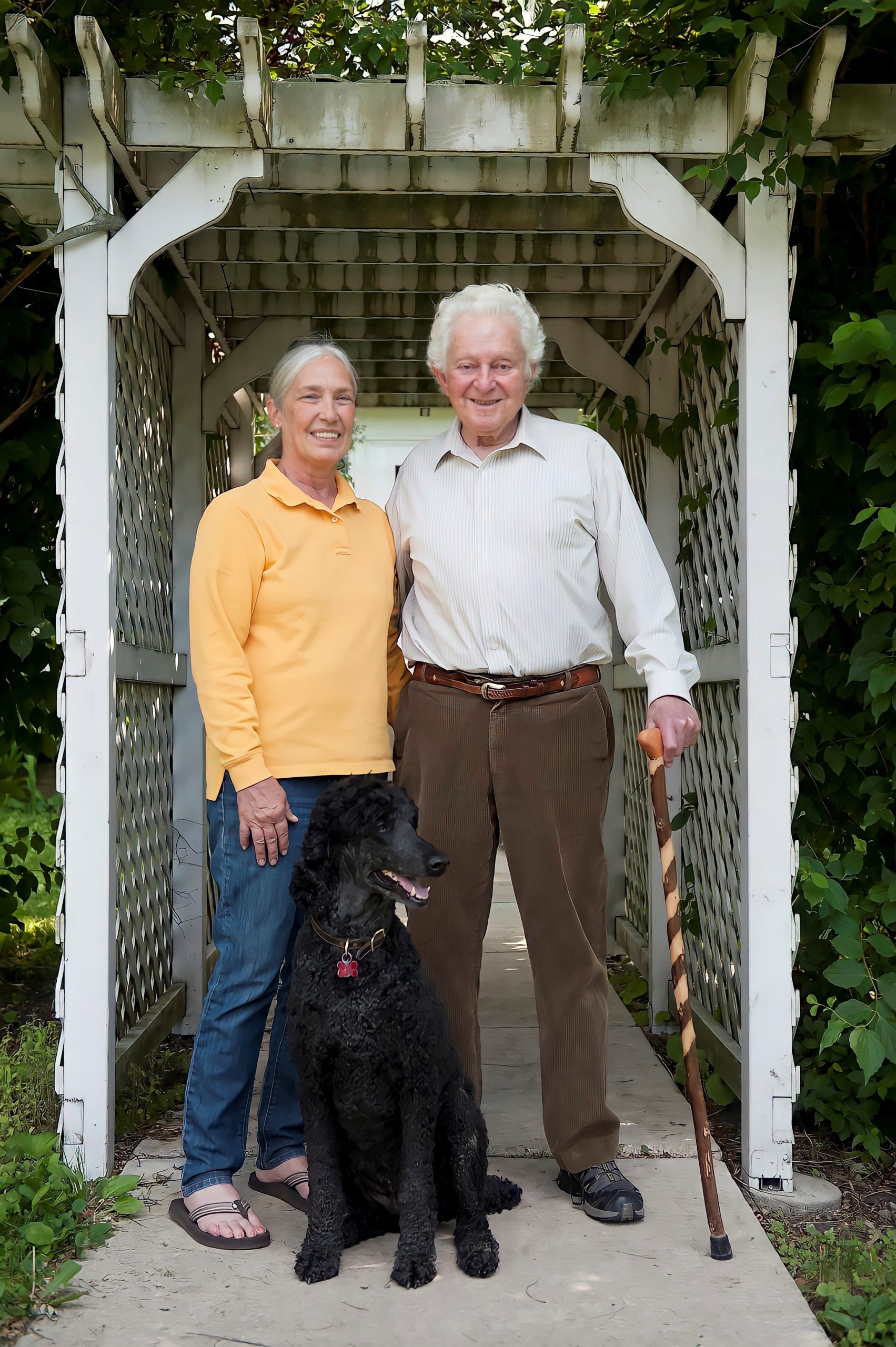
Lederman retired permanently from Fermilab in 2012. That same year, researchers at the European Center for Nuclear Research (CERN), deploying the Large Hadron Collider, detected a particle resembling the theoretical description of the Higgs boson — Lederman’s “God Particle.” Subsequent research has continued to verify the match of this particle with the theoretical model, and the mystery particle is continuing to behave as described in the Standard Model of physics, and as predicted by Leon Lederman.
In 2015, at age 92, Leon Lederman and his wife, Ellen, auctioned off his Nobel medal, raising $765,000 to finance his long-term care. The Ledermans spend most of his remaining years at their home in Idaho, where the great physicist enjoyed the company of his horses, cats, and dogs. Leon Lederman died at the age of 96.
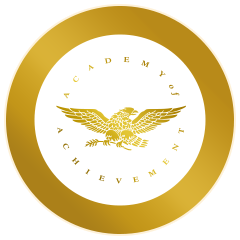
“I went into physics to hang around with the bright kids. I wasn’t doing anything else, and I didn’t want to look dumb, so I thought I’d pretend to be a physicist, just like the others. It was five or ten years after my Ph.D. before I realized I was pretty good.”
Leon Lederman’s modesty belied the scale of his achievements. In his lifetime, he was recognized as the world’s foremost experimental physicist, one of the very small group of scientists who have revolutionized our understanding of the subatomic world.
In the late 1950s and early ‘60s, he participated in the discovery of the K-meson particle and the non-conservation of parity during muon decay. In 1962, with Melvin Schwartz and Jack Steinberger, he designed and performed an experiment that proved the existence of the muon neutrino, an effort that eventually earned the three scientists the Nobel Prize in Physics. He led the efforts that found the first anti-matter particle in 1965 and the bottom quark in 1977. As director of the Fermi National Accelerator Laboratory in Batavia, Illinois, he laid the groundwork for the discovery of the mysterious top quark. After stepping down as director, he continued to write and teach, and campaigned vigorously to improve science education in the United States.
Speaking to someone who knows nothing about physics, what turns you on about it?
Leon Lederman: First of all, let me say that beauty’s in the eye of the beholder. People are turned on by many things in science, or in humanities, or any serious study. As an early graduate student, when it’s the time to choose your field of research, you make an important decision. I was committed to physics, but physics has many subfields.
I had spent three years in the Army, and the first year in graduate school’s a tough one because I had forgotten how to study, and I wasn’t doing that well, and the classes were very crowded. The professors were just getting back from their own war work and didn’t have much time for counseling. And so I was sort of at loose ends and depressed, and my coursework was poor. And I went around looking for my old college friends — who were either in graduate school or already had graduated — to get support, and they supported me. I remember trying to — several of them were clustered up at MIT, and they said, “Why don’t you transfer here, and we’ll help you?” So I tried to, but my early grades were so bad I couldn’t get into MIT. People at MIT are a little embarrassed about that now.
Then I had to make a decision. Which subfield to go into? There was nuclear physics. There was what’s called physics of materials. There was atomic physics, where you studied atoms. The guy who invented the laser was one of the professors at Columbia, and he was working on fields that had to do with that kind of research, which was exciting. There were so many fields, it was like being a kid with his nose pressed against the glass of a candy store, looking at all these candies and saying, “What’s fun?”
One of the fields was a brand-new field, which had to do with what you would call an atom smasher and we’d call a particle accelerator. Columbia University was building a large atom smasher off-campus. But they were building one which, when it was in operation back in 1951, was the largest atom smasher in the world, for a short time, before somebody else built a bigger one. That field was brand new to Columbia. I was intrigued by that, that I’d be almost as up on the field as the professors who had determined to bring that subject to Columbia but were not experts. That was exciting because it was a new field.
It was a totally new field. It had to do with “what’s inside.” That’s the title of it: “What’s Inside.” We know that if you take a small piece of chalk or any material and you start cutting it, you cut it into smaller and smaller pieces. If you keep cutting it and pretend that you can keep on doing this, eventually you get down to something which we call a molecule. This might be a molecule of some kind. Then we notice that a molecule is made of atoms, which are even smaller. It’s kind of a zooming down.
Now you’re getting into sizes which the human eye can’t see. So you go molecule, you go atom — and then inside the atom, it turns out that the atom itself is made of nuclei and electrons around it. That part of the field was well known. We were continuing that field into the nucleus of the atom. So you go zooming down, down, into even smaller dimensions. Like, if this whole room were an atom, then in the middle of the room, there’s a grain of dandruff. That’s the nucleus. You zoom down to the grain of dandruff, you look at it, and you say, “What’s inside?” So it was the added business of search, of trying to understand the basic building blocks, which to me was the turn-on.
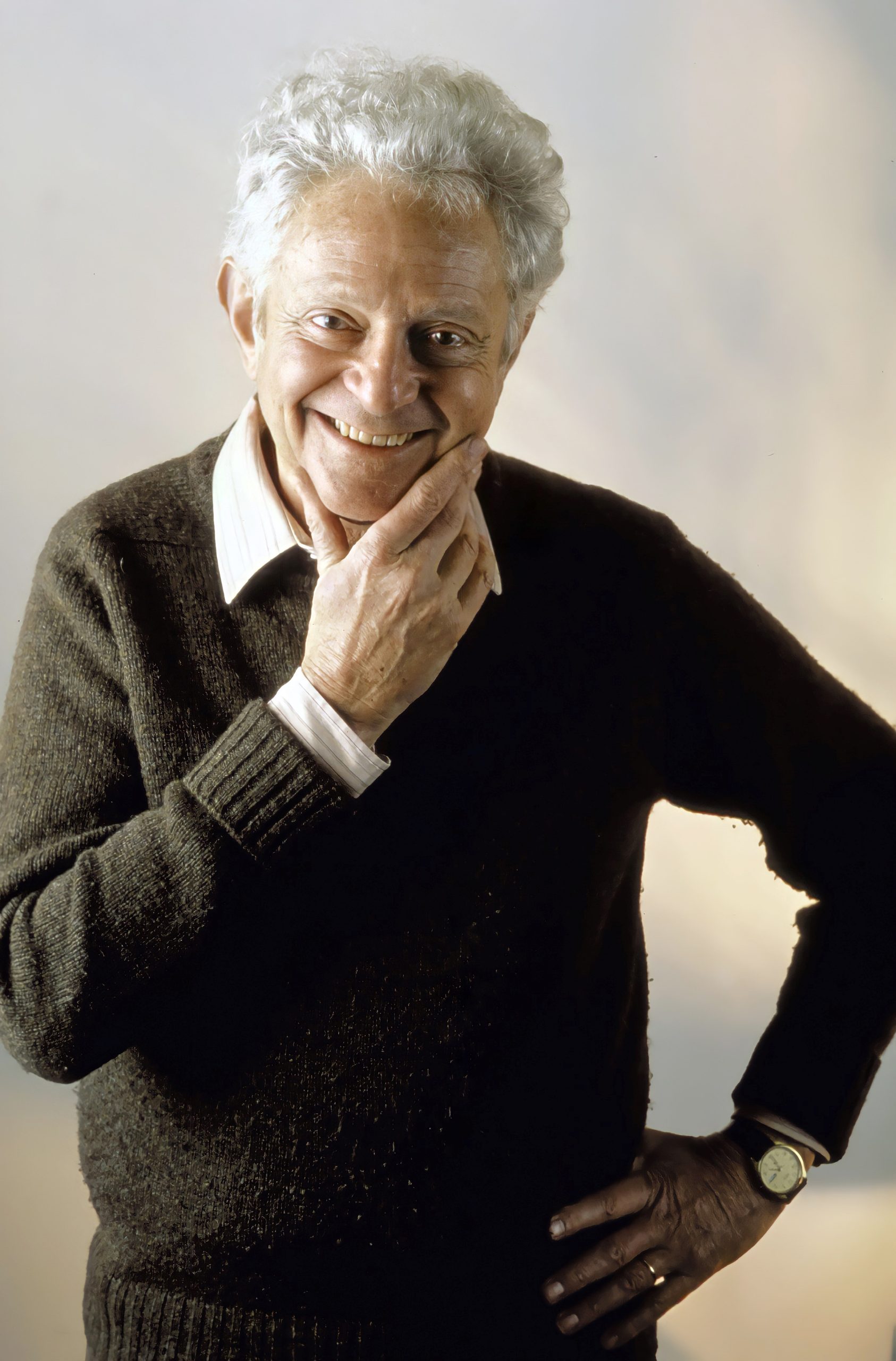
You eventually came down to what you yourself have described as “just barely a fact.”
Leon Lederman: That’s a particle that I have a great deal of affection for. That’s an answer to a question that goes, “How come you won the Nobel Prize, and what did you win it for?”
Here we go. What is a neutrino, and why did you win the Nobel Prize for discovering one?
Leon Lederman: We don’t have a blackboard here, and without a blackboard, a professor like me feels totally insecure. I don’t even have a little piece of blanket I can hold against my cheek under these circumstances. But let’s go anyway. What the heck!
We learned about what’s inside. Inside the nucleus, there are protons and neutrons, two of the constituents. Don’t panic; they’re all right. They don’t hurt. We looked inside those, too. But in the course of this kind of study, we found, for example, something called “radioactivity.” You’ve heard about radioactivity. It turns out that radioactivity always involves a mysterious particle which escapes. When you see something radioactive in our research, it’s a kind of explosion. A particle explodes and gives rise to other particles. You study these particles that come off from the explosion, and you reproduce what the event was.
Now, there are certain guiding principles in all of this that help us. One of them is the conservation of energy. It says that the total amount of energy should stay the same in any process. Like, if you put 14 people in a room, they can interact with each other, they can yell at each other, scream at each other, but hopefully, at the end of the day, there are still 14 people. The number of people is conserved, in that sense. In the same way, energy — if you keep track of it — it should balance. And in these reactions, it didn’t balance. Something was missing.
For a while, physicists jumped out of second-story windows. They got very upset because they really loved conservation of energy, and it looked as if they were going to lose it as a principle. Until somebody said, “Maybe there’s a particle escaping. Let’s assume it is, and since it doesn’t show up in our apparatus, it must be electrically neutral, and because it doesn’t show up for other reasons, it must be very small. So they used the diminutive ending “ino,” which is Italian for “little, little guy.” So a neutral, little particle.
And because of other properties, It became a very mysterious particle head. It was like, “Little fly upon the wall, ain’t you got no…” Anyway, I’m not going to finish that. But the neutrino had no electric charge. it turned out that its mass was almost zero, if not zero. Even today, in 1992, we think the neutrino may have zero mass, or if it has any mass, it’s a teensy, weensy amount of mass, not much. Lots of particles are detected because they make collisions. A proton hitting into some piece of lead, three inches thick, will never get through the lead because it will hit something, some other nucleus, and be stopped. Neutrinos didn’t have this strong ability to collide, so it didn’t seem to have any forces. So here it is, almost not even a thing. It had no charge, no mass, no strong force, and yet, we knew it was robbing energy from reactions, and it was very important. As we got to know more and more about it, it became crucial.
For example, why does the sun keep shining? The sun has been around for four billion years, and there was no mechanism which would keep it shining unless neutrinos were involved. So, whereas it became harder and harder to come to grips with the reality of neutrinos, conceptually, it kept taking an increasing, important role in our understanding of important processes like the sun shining, like radioactivity. In the late 1950s, the neutrino was becoming an increasingly irritating concept, which we had to come to grips with. It was confusing us. There was data that was contradictory; it didn’t make any sense. There were reactions that should have taken place but didn’t take place. That’s when a group of us at Columbia came on the idea that we should actually see, try to detect, neutrino collisions.
Now that’s a hard job because, if you try to calculate, using the best information we had, how much material — let’s say steel. How thick a steel wall do you need if a given neutrino coming into the steel wall should have a very good chance of never getting out? How long does that steel wall have to be? Ten feet, a hundred feet, a mile, ten miles? Turned out the answer is a hundred million miles. So we went to the authorities, and we said, “We need a hundred million miles of steel. We want to catch neutrinos.” No, of course, we didn’t do that! We thought more clearly, and it turns out, if you have two neutrinos, you only need half that thickness. And if you have a billion neutrinos — or a billion, billion neutrinos — then you might need a kind of detector that you could think of building. It would still have to be very massive and detailed. It turned out that we hit on a way of doing this with a detector, which, for that time, the 1960s, was very massive. It was ten tons of material, and it’s not just ten stupid tons of steel sitting there. You had to look inside to see the collision. So it had to be, in some sense, semi-transparent.
Anyway, the experiment was wildly successful. We discovered, in fact, that they weren’t one neutrino, but there were two kinds of neutrinos, and that’s what was giving us all the confusion. The number of neutrinos was doubled. And that, these two types of neutrinos, really set us on a road towards what we now call the “Standard Model,” a compact summary of all of this data that I’ve been telling you about — the data — lots of data that came out in the laboratories all over the world since 1960. So it became known as the “two-neutrino experiment.” Of course, when you tell somebody who’s not a scientist about two neutrinos, they say it sounds like an Italian dance team. “Ladies and gentlemen, we now have the Two Neutrinos!”
That’s the story, and eventually, it came to the attention of the King of Sweden, and we were invited to a great party in Stockholm.
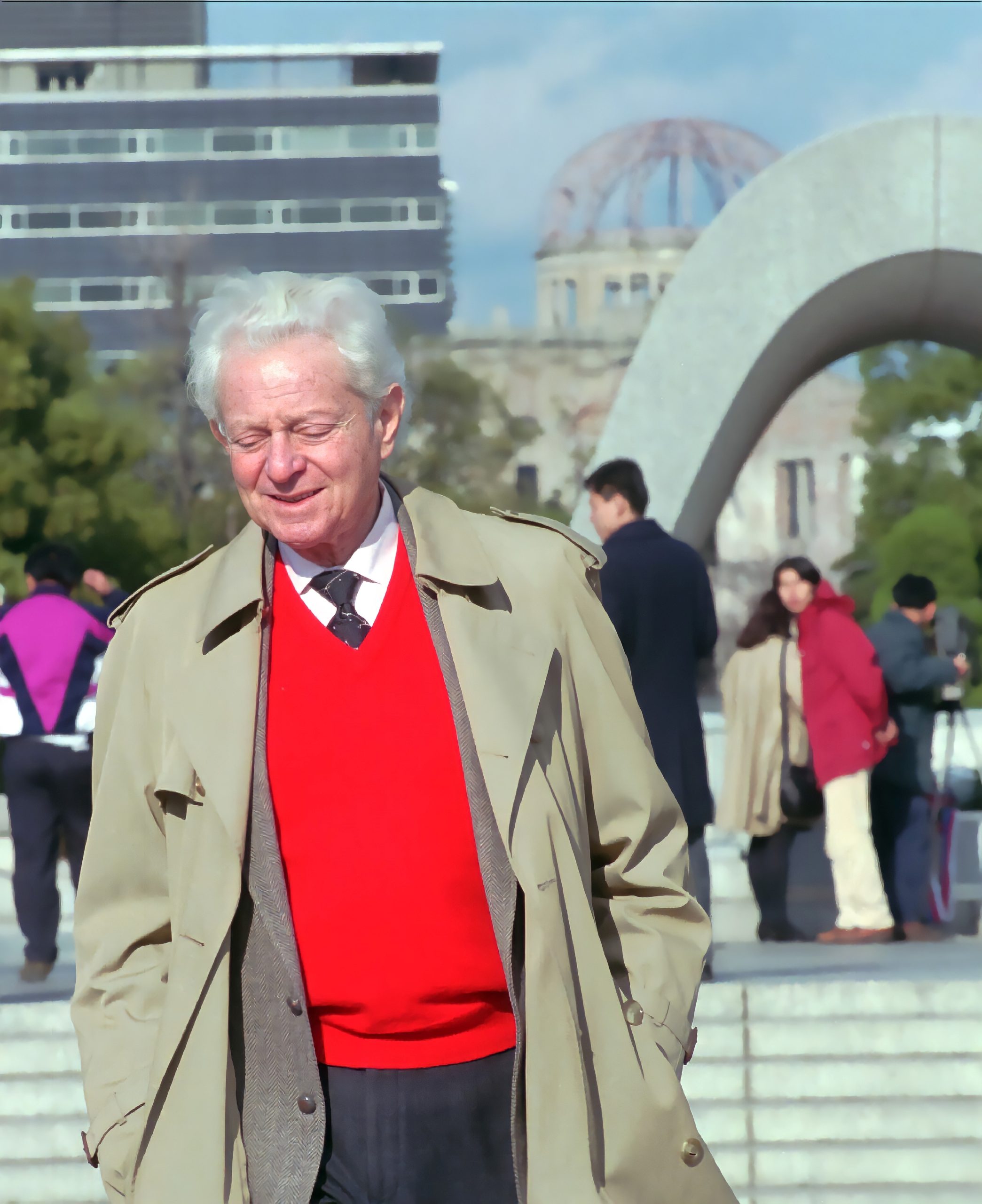
When you won the Nobel Prize, was that a surprise? Where were you? How did you react?
Leon Lederman: Because of the time change, you’re generally notified about this at five or six in the morning. They assume they’ll be forgiven for waking you. Was it a surprise? Not exactly. It’s always a surprise, but I knew I had been nominated. My kids used to tease me whenever the physics Nobel Prizes were announced and I wasn’t included. They would say “Uh huh, another year in which you didn’t win it.” And I would say, “ I’ve done so many things, they can’t decide what to give it to me for.” That was my standard joke. So I was relaxed. I didn’t worry too much about it because I was a senior, full professor; I’d gotten lots of awards and recognition. It would be a little icing on the cake. It’d be nice, but I didn’t think too much of it.
Then you get this call at six in the morning. My wife picked up the phone and said “Yes, yes, yes, he’s here,” and handed me the phone and muttered something which I won’t repeat because she knew our life would change, at least for a while. It was a gentleman in Sweden notifying me about this award. And you laugh. As soon as I hung up, we started laughing. It was a great, great emotion. About eight minutes later, it got on the wires, and we started getting calls. About a half-hour later, the first friends arrived for champagne. So it was a little party, but it changes your life more than I would have expected.
How so?
Leon Lederman: What I didn’t expect was the awe with which people treat this thing. You know, I thought it’s another prize, great. I’d like to have it, and there’s even a check that goes with it. Wow! That’s great. A nice party you go to — almost all expenses paid except for my wife’s dresses. But it’s more than that. It really has an aura about it. First of all, you become an expert on everything. You get interviewed for, “What do you think about the Brazilian debt, or social security, or women’s dresses?” Well, there I had an opinion — but the other things, I don’t know — as short as possible! And that’s part of it — and that, you’d better be careful about. But it turned out that if you ever want to do anything in the way of education, for example, or science policy, where you want to change laws or move people to be active — boy, then having a Nobel Prize helps a lot! You get into places that normally would be very difficult to get into.
You’ve spoken a lot in your writings about the joy of discovery. What does it feel like? Can you recall some occasions when you knew you had something?
Leon Lederman: Almost all of them seem to happen at three o’clock in the morning, mostly on Thursdays. I don’t know. There is that occasion when you realize that you’re learning something that nobody else knows. It may be you’re alone. It may be you’re with a graduate student. It may be you’re with a colleague. Sometimes it’s very gradual. The data accumulates slowly, and there’s not what we would call the “eureka” instant. But sometimes it does happen that way. I can remember several examples of suddenly realizing that the world is very different from the way the four billion people or so that are on the planet know about it.
Can you give us an example?
Leon Lederman: Here’s an example. Once upon a time, we were studying a particular symmetry. Symmetry is very important. Simplicity is a key sort of force, forcing our intuition.
We really do have a fundamental belief that the world is very simple — that when we finally understand the universe, we’ll be able to fit it on a simple T-shirt with one sort of symbol and an explanation point: “This is the way the world works!” We’re not there yet. And one of the ingredients in simplicity is symmetry, and symmetry means what most artists know about and most people who appreciate Greek sculpture know about — it’s something which looks the same. You see a row of Greek columns, and you see that symmetry of the columns. Or you see a perfect Grecian figure, which is again, symmetric between the left and the right side. And we were testing one of these deep symmetry principles, which everyone believed was perfect. It’s like, if you have a perfect human being who’s perfectly symmetric, and half the person shows up, like one of these magic boxes you sometimes see, say, from just right down the middle. You see the left side, and you say, “I can deduce what’s behind the screen, even though I don’t see it.” By looking at the left side, I know what the right side looks like. So you can make predictions about nature from your belief in symmetry.
We were testing this idea. And we had an idea, which we got on a Friday evening, for how to test it. And it turned out that some of the apparatus that we needed was already there on the floor of the accelerator, being polished by a graduate student who was going to use this particular apparatus to do his thesis.
Where was this?
This was at Columbia University, at this new atom smasher, which by that time was well-honed and well-used. We got the idea, actually, during a Chinese lunch in the city. The physics department at Columbia has Chinese lunches and lots of animated conversation between slurps of winter melon soup and hoisin and all the good northern Chinese food we like to eat there.
Suddenly it became clear that there was a way of testing this parity idea. And so, we went to the laboratory and dashed in on this poor, confused steward and started rearranging the apparatus and telling him, “Do this, do that, do the other thing,” and he saw his thesis flying out the window. “What are you doing to my apparatus?” And someone said, “Don’t worry about it. It’s going to be great.” And we worked on the weekend, preparing this experiment. And it turned out that we started collecting data Monday evening, and by three o’clock Tuesday morning, we knew something that nobody else in the world knew: that this symmetry idea that we had been working on was not a perfect symmetry, that there was an imperfection in the symmetry, a very important imperfection in the symmetry. That was the key discovery. That’s the eureka moment, when suddenly you know something. Your hands sweat; you get into all kinds of symptoms of tremendous excitement. First of all, it’s fear. Is it right? And it’s incredible humor. “How could it be any other way? It had to be that way! How could we have been so stupid not to see this?” The next thing is, “When can I tell people?” and “Who do I want to call first?” Now, all these things jumble in on you in a great feeling of tremendous excitement. Of course, many scientists say, “I do science because I’m curious.” That’s not enough because if you were satisfying your own curiosity and you couldn’t tell anybody how clever you are to find it out, it would be useless. So you’ve got to communicate. All of this piles in, in this moment of discovery.